Abstract
This article offers a deep analysis of activating streptokinase which looks into the biotechnological challenges and future perspectives. One of the main problems associated with streptokinase is its short half-life, complex production process, and immunogenicity which hinders patients from getting a successful reperfusion. Yet, the development of biotechnology methods that would not face these drawbacks, such as the strategy of recombinant streptokinase production and enzyme engineering theories, is a promise. This paper aims to provide an overview of biotechnological breakthroughs in the production of streptokinase with a focus on discovering long-lasting formulations exhibiting less immunogenicity and in addition the economic viability and scalability of biotechnological production processes. This article also gives the comping image of known purification methods like chromatography, ultrafiltration, and I-A chromatography for the separation and purification of streptokinase. In addition, the author presents the advantage of such suitable production technologies as cell-free production systems and E. Coli system optimization, such as recombinant streptokinase synthesis in Yeast systems. Biotechnology is turning out to be a new area, having a future boom due to the rising innovation and demand that are associated with the prospects revealed by the market trends.
keywords
Streptokinase, Enzyme Engineering, Recombinant Streptokinase, Streptokinase Gene Cloning
Introduction
Streptokinase Overview and Medicinal Applications
Streptokinase is a naturally occurring protein,
many strains of hemolytic Streptococci, secrete it (Deepika, 2009). It was the first drug introduced to myocardial infarction 40 years ago and is a main fibrinolytic agent and has been a part of the WHO Essential Model List of Medicines. Streptokinase is a thrombolytic drug (Ehab, 2022) and a potent plasminogen activator (Pimienta, 2007).
Streptokinase is a proteolytic enzyme and is derived from Group C beta-hemolytic streptococcus bacteria. It activates the fibrinolytic system and a complex is formed with plasminogen. It converts plasminogen to plasmin and dissolution of thrombus occurs as insoluble fibrin is lyzed. Streptokinase administered intravenously has proven to be effective in the survival of patients with acute myocardial infarction (Battershill, 1994; Battershill, Benfield & Goa, 2012). We can get more improved results when aspirin is combined with streptokinase or when (rt-PA) accelerated recombinant tissue plasminogen activator. Left ventricular function is improved. It is considered as first-line therapy (Battershill 1994].
It is a thrombolytic drug that comes under the class of plasminogen activators with the other two urokinase UK and TPA Tissue-type plasminogen activators (Assiri, 2014). It is an extracellular protein with 440 amino acids; 26 N-terminal single peptide amino acids in total and is an efficient thrombolytic agent. (Aghaeepoor, 2019) Streptokinase has effectively treated Acute Myocardial Infarction AMI.
Pharmacological Properties
It is used to treat acute ischemic stroke in which reperfusion with thrombolytic agents therapy is used. There is a high risk of cerebral bleeding and reperfusion-induced injury in case of myocardial infarction. It is used in cardiovascular diseases treatment (heart attacks and stroke) and dissolves blood clots Treat various circulating disorders (pulmonary embolism, deep venous thrombosis, myocardial infarction, They restore blood flow by converting inactive plasminogen into active plasmin which works by dissolving clots and degradation products become soluble which are engulfed by phagocytosis.
It gives its medicinal application by methods like plasminogen conversion to plasmin, fibrinogen factors 5 and 8 depletion, and alpha 2 anti-plasmin level reduction. Microcirculation is improved and it helps in the reduction of infarct size and inhibits neutrophil and platelet aggregation. Intravenous dosage of about 1.5 million units infused over 30 or 60 minutes. Adverse effects may be hemorrhage, allergic reaction, transient hypotension, or bradycardia (Battershill, 1994).
Importance of Biotechnological Methods in Enhancing Streptokinase Production
Streptokinase was synthetically prepared by the streptokinase gene and cloned with Pet21B plasmid for periplasmic expression. High yield was obtained by using the shake flask method. It is the most cost-effective industrial production method so far. Streptokinase production was enhanced by using the Fed-Batch culture strategy in which Recombinant Escherichia coli was used and by controlling some factors like DOC Dissolved Oxygen Concentration, nitrogen sources, and pH control with no effect on cell growth and this increased production was directly proportional to plasmid segregational stability. It was found that SK production was increased 14 folds (1120mg I-1 protein0)
Natural SK has antigenic potential which is combated by using rSK recombinant streptokinase which is also proven to be a safe and effective thrombolytic agent. APSAC Anisoylated Plasminogen Streptokinase Activator Complex is a more efficient intravenous formulation of 30U dose over 4 to 5 minutes rather than streptokinase intravenous infusion and treat myocardial infarction. It has only selective action on thrombi fibrinolysis. It has the advantage of easy administration and 10 times greater thrombolytic potency (Monk, 1987).
Purpose and Scope of the Review Article
Natural hosts yield a very small quantity of streptokinase and it's quite difficult to meet the increase in the need for clot-dissolver drugs due to thrombo-embolic disorders. So, recombinant DNA technology should be breached.
Historical Background of Streptokinase Production
Early Methods of Streptokinase Extraction and Purification
Highly purified streptokinase was prepared by 2 methods: (Renzo, 1967)
? Column chromatography on diethylaminoethyl
? Column electrophoresis in a sucrose density gradient
Specific activity was found to be constant when preparations were chromatographed at least twice on diethylaminoethyl cellulose and depending on gel electrophoretic and ultracentrifugal analyses they appear to be monodispersed. Molecular weight was found to be 47,600 when determined by equilibrium sedimentation, and when treated with 0.1 M phosphate buffer at pH 7.5 with 5 M guanidine hydrochloride / 8 M urea, the sedimentation coefficient was lowered without changing molecular weight. When amino acid analysis was done it was found that streptokinase consists of a single polypeptide chain having no subunits. Moreover, cysteine and cysteine were absent (Renzo, 1967).
Streptokinase has a fixed amino acid composition with formula Asp68 –Thr30-Ser24-Glu46-Pro20-Gly21-Ala23-Val23-Met3-Ile22-Leu40-Tyr20-Phe15-lys33-His9-Arg21-Try1. One molecule of protein contains almost 60 aspartic and glutamic residues. When preparations are most highly purified carbohydrates and phosphorus are not present and amino acids (esters, naphthyl esters, acetyl-tyrosine ethyl esters) which are basic in nature, when used as substrates inactivate the preparations (Renzo, 1967).
Challenges Faced in Traditional Production Methods
Traditional methods of production of therapeutic enzymes pose many challenges like strain instability, cost-effectiveness, lack of infrastructure, and due to which there is insufficient production. There is a high demand for thrombolytics due to rising cardiovascular diseases, so we need genetically engineered strains and fermentative methods to overcome challenges faced in the production of therapeutic enzymes like Streptokinase, Urikase, etc. (Ghosh, 2021).
Need for More Efficient and Scalable Production Techniques
There are many factors that affect the efficacy of streptokinase production so we are in search of the recombinant source of a therapeutic protein. Efficient production is needed for the following factors: (Pimienta, 2007).
1. Improve low yield
2. Lack of developed gene transfer methodology
3. Pathogenesis for natural host
? Streptokinase can be prepared by the Gram-positive bacterium Streptomyces lividans (expressed and secreted). The encoding structural gene of Streptokinase SK was fused with one of the 2 signal sequences (Pimienta, 2007).
1. Streptomyces venezuelae CBS762.70 subtilisin inhibitor (vsi) signal sequence
2. Streptomyces lividans xylanase C (xlnC) signal sequence. Both these are translocated by following pathways: (Pimienta, 2007)
1. Vsi protein by Sec pathway
2. XlnC protein by twin-arginine translocation (Tat) pathway
Among the above pathways, translocation and expression of streptokinase equisimilis ATCC9542 skc-2 in Streptomyces lividans proved to be effective in the following ways:
1. Src-dependent pathway produced a 30 times greater yield but both pathways (Src-dependent and Tat-dependent)performed successful translocation
2. Lab-scale conditions of yield of secretory SK were improved four times when small-scale fermentation was used
3. Streptomyces lividans has been proven as an efficient host for biopharmaceutical product bio-active form production worldwide (Pimienta, 2007).
Mature SK Streptokinase protein was prepared by synthetic gene encoding in which mRNA secondary structure and codons were cloned and then they were expressed in vector pET-3a and Escherichia coli BL21 (DE3) was formed. IPTG induction methods were employed to optimize native SK protein expression. Rapid dilution method was used for refolding of recombinant SK but first, non-soluble rSK inclusion bodies were purified and 6M guanidinium chloride was used for enzyme denaturation, and then was purified by anion exchange chromatography technique and ELISA was used to evaluate its molecular identity and conservation. The clot lysis efficacy and enzymatic activity of purified recombinant streptokinase rSK protein was found to be 1.945 x 106 IU/mg with 62.94 ± 2.3%. So this was the cost-effective industrial production (in-vitro thrombolytic activity) method of enzyme which gave a high yield rSK production. This method had a similarity with commercial SK.
Many genetically engineered products are being made to reduce immunogenicity and improve fibrin specificity. It is done by employing minor changes in native streptokinase at two sites (amino and carboxyl-terminal). These changes had a direct impact on fibrin dependence and activity.
Biotechnological Approaches for Streptokinase Production
Recombinant DNA Technology
Gene Cloning and Expression of Streptokinase
In research performed by Horst Malke* and Joseph J. Ferretti, Using bacteriophage K and E. coli plasmid vectors, the streptokinase gene (skc) from S. equisimilis strain H46A was successfully cloned in E. coli. The generated streptokinase is detectable extracellularly and in the periplasmic region, indicating the possibility of active secretion. It also maintains its substrate specificity. Understanding plasminogen activation and gaining knowledge about virulence genes in streptococci using recombinant DNA techniques are two benefits of streptokinase gene cloning (Malke, 1984).
In another research, Pichia pastoris is a promising alternative host system since it was able to produce steady expression levels of around 77 mg/liter when the streptokinase gene was cloned and expressed. This system is in line with recent developments in second-generation plasminogen activators and fusion proteins for improved fibrin specificity. It allows for additional research on streptokinase functional domains, plasminogen interactions, and the creation of genetically modified molecules with enhanced therapeutic potential (Hagenson, 1989).
In an experiment performed by researchers in India, streptokinase (SK) was cloned and expressed in S. cerevisiae using the GAP promoter from P. pastoris. After screening S. cerevisiae transformants, a clone expressing SK at 1050 IU/mL was selected and production of SK was significantly increased to 2352 IU/mL with further adjustment of growing conditions using Plackett-Burman design and response surface methodology (RSM). The recombinant SK's biological activity was validated by functional tests. The study presents a viable strategy for employing S. cerevisiae to produce functional streptokinase on an industrial scale (Vellanki, 2013).
Advantages and Limitations of Recombinant Techniques
Advantages
In clinical trial studies, It has been demonstrated
that recombinant tissue-type plasminogen activator (rt-PA) exhibits selective targeting, a decreased risk of bleeding, and strong thrombolysis-generating efficacy producing remarkably low rates of hospital mortality and morbidity, proving that recombinant technology improves patient outcomes for thrombolytic therapy. (Vellanki, 2013).
Recombinant DNA technology guarantees large-scale production and distribution, which is essential for efficiently satisfying medical demands. Furthermore, the remarkable specific activity and purity of r-SK (8.9x10^4 IU/mg and 95.7%, respectively) demonstrated the quality and precision attained. Additionally, by eliminating the signal peptide sequence and enhancing fermentation conditions, stability was increased, guaranteeing dependable and consistent output (Sohaimy, 2011).
Another benefit is cost-effectiveness, which is demonstrated by the purification process use of ion-exchange chromatography, which is less complicated and less expensive than monoclonal antibody chromatography. Recombinant technology simplifies a process that lowers cycle time and boosts overall efficiency, making it extremely scalable and industrialization-ready. Strict quality control procedures, such as the capacity to efficiently regulate fermentation conditions, also help to sustain high r-SK yields while reducing the synthesis of undesirable proteins, guaranteeing stable product quality (Zhang, 1999)
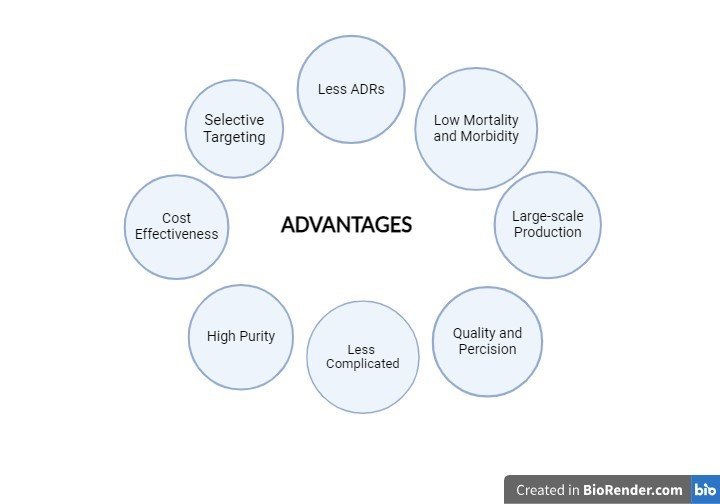
Figure 1
Figure 2
Limitations
Recombinant technology requires proficient knowledge of protein biochemistry and molecular biology. Because safety and quality control are major concerns, the manufacturing of recombinant proteins, particularly for medicinal reasons, is closely supervised by regulations. Recombinant proteins may occasionally show immunogenicity, which may affect patient compliance and adherence. Certain research or industrial contexts may be limited by the substantial initial investment required to set up a recombinant protein manufacturing system in terms of infrastructure, equipment, and qualified workers (Sohaimy, 2011).
Streptokinase's propensity for rapid degradation may have an impact on its long-term stability and biological activity. The initial specific activity could not be adequately determined due to the incompatibility of the crude culture medium with some techniques, such as the chromogenic substrate assay (Pimienta, 2007).
Pharmacovigilance of recombinant DNA technology is limited due to inadequate reporting of adverse drug effects and lack of data. The subjective assessment of severity could have an impact on the accuracy of a classification. Multivariate analysis is challenging because of small sample numbers and other real-world issues. It is also difficult to discover conclusive proof of some side effects, and maintaining tight regulation and quality control for SK products is still a challenge (Betancourt, 2005).
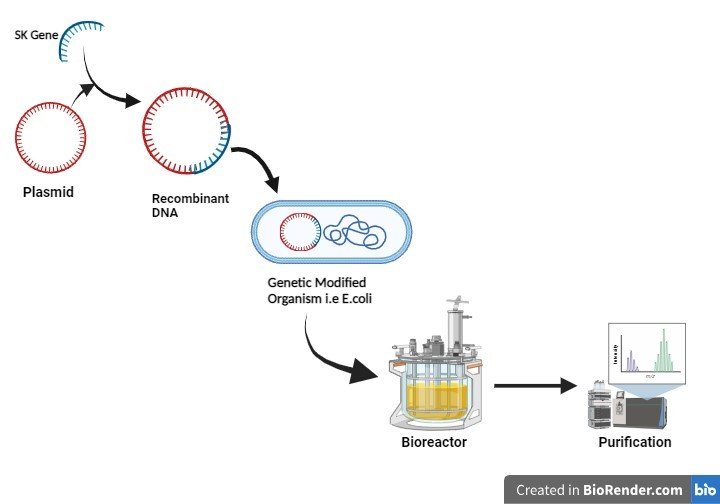
Figure 3
Enzyme Engineering
Rational Design
The rational design approach to enzyme engineering predicts possible mutants based on knowledge of the connections between protein structure and function (Longstaff, 2005). Using oligonucleotide-directed mutagenesis, histidine, glutamic acid, or alanine were substituted for streptokinase's glycine-24. Histidine or glutamic acid substitutions nearly completely eliminated streptokinase activity, however alanine substitutions kept streptokinase active. While streptokinases containing histidine-24 or glutamic acid-24 linked to human plasminogen properly, they were unable to produce active plasmin. In contrast, wild-type streptokinases containing alanine or glycine-24 could produce active plasmin. The findings suggest that the activity of the human plaminogen-streptokinase complex depends on the short, uncharged alkyl group side chain on the 24th amino acid residue of streptokinase (Lee, 1989).
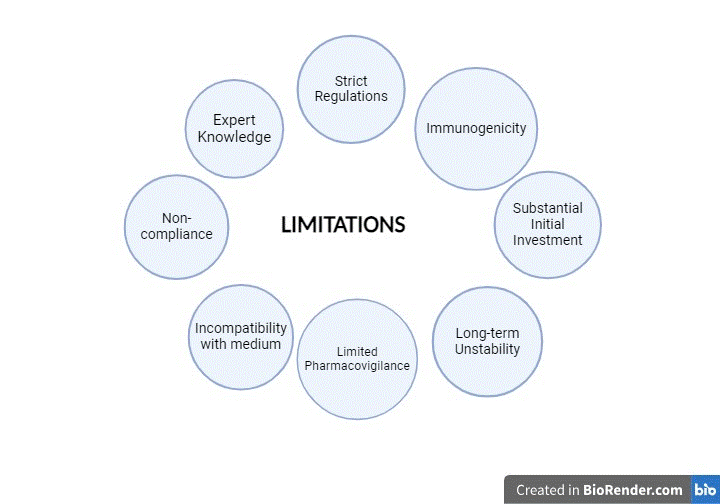
Directed Evolution
Directed evolution is a two-step, iterative process that starts with the creation of a library of variants of a biological entity of interest and ends with a high-throughput screening of this library to find mutants with improved characteristics like increased activity and selectivity.
According to a study by scientists at the University of Agriculture, Faisalabad, introducing random mutations into a microorganism's genome is a useful method for increasing streptokinase output. The most noteworthy outcome of this investigation was the development of the extremely effective S. agalactiae EBL-32 mutant and the improved fermentation technique for streptokinase production.
Improving Streptokinase Stability and Activity
The primary issues with the enzyme are its short half-life in vivo, which necessitates higher dosages and increases adverse effects, and the immediate immune response it elicits. Several carrier materials were looked into in previous years for streptokinase immobilization, to get past the difficulties listed above.
Nanoparticle Mediated Encapsulation, Entrapment & Cross-linking
In research performed by Iranian scientists, Magnetic nanoparticles coated with silica (SiO2-MNPs) were employed as delivery vehicles for the SK. These nanocarriers increase the enzyme's stability and produce a shorter duration of action relative to native SK resulting in the prevention of hemorrhagic side effects of the enzyme (Adayon, 2015).
In another study, chitosan (CS) nanoparticles loaded with streptokinase were developed, resulting in improved Streptokinase loading efficiency onto CS nanoparticles by adjusting formulation parameters including pH and CS and streptokinase concentrations. According to dynamic light scattering (DLS) and scanning electron microscopy (SEM), the nanoparticles had a homogeneous and spherical morphology. In vivo tests in rats showed sustained activity over time compared to native streptokinase, suggesting extended circulation and improved stability (Modaresi, 2023).
In 2017, poly(lactic-co-glycolic acid) (PLGA) nanoparticles were used to entrap streptokinase using the electron spray method. The optimization of several parameters influencing the size and dispersion of nanoparticles was done. While SK activity was significantly reduced after electrospray, with just 19.2% of activity conserved, encapsulation efficiency was good at 90% (Yaghoobi, 2017).
Drug Delivery Using Vesicular System
When compared to free streptokinase, liposomal entrapped streptokinase demonstrated noticeably better clot lysis in an experimental rabbit model. Additionally, liposomal streptokinase was still effective in rabbits that had received an anti-streptokinase vaccination, suggesting that it may be preferable to free streptokinase in patients who have anti-streptokinase antibodies (Perkins, 1997).
The synthesis of tissue plasminogen activator (TS) liposomes containing acylated-RGD (Arginylglycylaspartic acid) peptide and DOPE (dioleylphosphatidyl ethanolamine) improved drug entrapment efficiency (~18%; upon interaction with activated platelets, over 90% of the drug was released in a regulated manner in vitro). TS liposomes demonstrated a notably greater (p < 0.001) thrombolytic activity in thrombolysis experiments when compared to non-liposomal SK. This was explained by the drug's immediate release at the clot site upon engagement with activated platelets, which extended the drug's duration of action (Vaidya, 2016).
Plant Virus-Based Nanoparticles
In a study by American scientists, tobacco mosaic
virus was mutated and tissue plasminogen activator (tPA) was built into mosaic virus particles. It was demonstrated that the particles could bind and disintegrate blood clots in vitro. In an ex vivo thrombosis model, they showed improved thrombus localization and effectiveness with the prevention of internal bleeding in comparison to free tPA (Pitek, 2017).
In another study performed by scientists at Cleveland and California, tissue plasminogen activator (tPA) bioconjugated onto TMV nanoparticles, and an estimation of tPA loading efficiency on TMV was made. Characterization verified that tPA and TMV were covalently bound and that tagging with a fluorophore (sCy5) was successful. TMV-tPA formulations were found to have thrombolytic efficacy and safety in both in vitro and in vivo studies, suggesting that they may have advantages over free tPA (Pitek, 2018).
Fermentation Processes
Optimization of Culture Conditions for Streptokinase Production
In research performed in Iran, the culture conditions for streptokinase (SK) secretion in Escherichia coli were successfully optimized by using the Plackett-Burman design combined with response surface methodology (RSM). Triton X-100, glycine, and Ca2+ were shown to be the most efficient in increasing the membrane's permeability, which resulted in a seven-fold rise in SK secretion. Higher enzyme activity was the outcome of these components working together to facilitate SK's translocation over the bacterial membrane. This study offers important new information on how to best culture conditions to maximize the secretion of recombinant proteins (Aghaeepoor, 2017).
In another study, Streptococcus equisimilis production of streptokinase is increased as a result of UV mutagenesis. With Baewald medium, the yield was maximum. SK production was optimum at 1% glucose, while activity was enhanced at 2.5 g/l sodium chloride in the medium. The ideal parameters were 37°C and pH 7. The strain improvement achieved through UV-induced mutation demonstrated potential for low-cost, large-scale streptokinase manufacturing (Devi, 2020).
To optimize the synthesis of streptokinase in P. pastoris, a constitutive expression vector containing the GAP promoter was constructed. Response surface methodology indicated that under ideal conditions—2.90% dextrose, 2.49% peptone, pH 7.2, and 30.4°C temperature—streptokinase production would increase by 95%, reaching 2,136.23 IU/ml. The modified medium's effectiveness was validated by validation tests, which set the stage for the large-scale manufacture of streptokinase (Vellanki, 2013).
Use of Microbial Hosts for Large-Scale Production
In 2007, Optimal fermentation conditions allowed for the large-scale synthesis of streptokinase (SK) in Streptomyces lividans. The best yield was achieved by combining SK with the Sec-dependent Vsi signal peptide. A number of procedures, including ammonium sulfate precipitation and DEAE-Sephacel column chromatography, were used to purify SK from the culture supernatant. With a specific activity of 2661 IU/mg protein, it was discovered that the isolated SK exhibited biological activity. The yield of bioactive SK in S. lividans is predicted to increase with further improvement of the fermentation and purification methods, confirming its potential as a useful host for the production of biopharmaceutical products like streptokinase (Pimienta, 2007).
Advancements in Downstream Processing
Purification Techniques for Streptokinase
Chemical Reduction Method
Chemical reduction was the approach employed in
the early days to purify streptokinase. A streptococcus strain was cultured in a fermenter with constant glucose feeding at the ideal temperature and the optimum pH. The fermentation product was sterilized using filtration and ultrafiltration techniques and the pH was adjusted and precipitation was then achieved using methanol. After removing contaminants and trace elements, the yield of SK obtained is 88% using this approach (Karimi, 2011).
Chromatographic Method
In 2008, Iranian researchers purified streptokinase using affinity chromatography. Affinity chromatography produced highly pure streptokinase with high specific activity, allowing for effective purification in a single step, with minimum streptokinase activity loss (5%) observed.[32] Ion affinity chromatography demonstrated a purity of 93.2%, surpassing the performance of hydrophobic chromatography (90.4%) and ammonium sulfate pre-treatment combined with affinity chromatography (88%). This demonstrates affinity chromatography's effectiveness as the last purification stage in the manufacturing of streptokinase, providing excellent purity and efficiency (Rahimi, 2020).
Figure 4
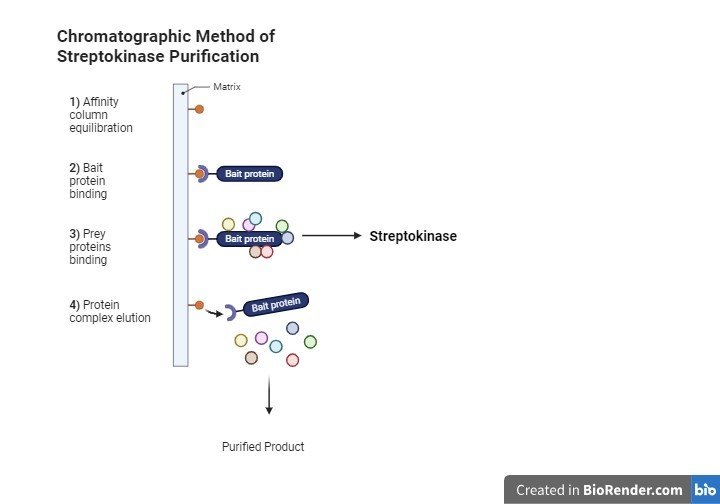
Formulation and Storage Stability of Streptokinase
Development of Stable Formulations
Streptokinase (SK) liposomal formulations were created with improved stability and extended in vivo circulation times. The freeze-thaw approach of incorporating SK into liposomes preserved its activity. The size and loading efficiency of EPC-based liposomes were ideal, and the inclusion of PEG lengthened their circulation time even further. All things considered, liposomal encapsulation extended the half-life of SK and provided the possibility of tailored distribution, indicating more extensive uses for short-lived proteins in vivo (Jin, 2015).
A stable formulation of recombinant Streptokinase (rSK) for hemorrhoid treatment was developed, incorporating sodium salicylate as an absorption enhancer and anti-inflammatory agent, thimerosal as a preservative, Span 60 as an emulsifier, and Witepsol W25 as a suppository base. The formulation, containing 100,000 IU per gram of suppository, was assessed for stability over 18 months under refrigerated conditions. Results showed that the formulation maintained its physical, chemical, and biological properties, indicating stability throughout the study period (Aguilera, 2013).
The table below shows marketed streptokinase products and their manufacturer.
Table 1
Brand
Name | Manufacturer |
Cardiostrep Eskinase Kabikinase Myokinase Niskinase Prokinase
Streptase | Kee Pharma Ltd. Dabur Pharma Ltd. Pharmacia
Healthcare Ltd. Biocon Ltd. Neiss Labs Pvt. Ltd. Emcure Pharmaceuticals Ltd. Streptokinase Bharat
Serums and Vaccines Ltd. & TTK
Healthcare Ltd. Sanofi Aventis Pharma India |
Long-Term Storage Conditions
Long-term storage stability of streptokinase (SK) standards was assessed through accelerated degradation testing, predicting potency losses of 0.018% and 0.006% per year at ?20°C for samples B and C, respectively, using the Arrhenius model. Reconstitution showed minimal potency loss (99.3% and 101.7% potency remaining for samples B and C, respectively) even after 24 hours at 4°C. Thus, both samples B and C are deemed suitable replacements for the third International Standard for SK, indicating excellent stability under various storage conditions.[36] According to ICH guidelines, unopened ampules should be stored in the dart at or below -20 ºC. Due to the inherent stability of lyophilized material, ampules should be shipped at ambient temperature.
Biotechnological Challenges and Future Perspectives
Streptokinase is a good and successful medicine for acute myocardial infarction disease due to its action over tPa or another drug. One of the notable cardiovascular illustrations of the role of thrombolytic trends in acute myocardial infarction can be made with the use of streptokinase. This treatment is a key agent that allows the dissolving of blood clots. Streptokinase is a thrombolytic agent that is derived from certain streptococci and specifically acts by catalyzing the conversion of plasminogen to plasmin. The vital role of this enzyme in the management of various conditions, including myocardial infarction and other complications attributable to thrombosis, is thus demonstrated. Although it possesses enormous practical benefits in the life of nonhuman beings, the limited shelf-life, manufacture, insufficient purification, stability, and immunogenicity are the main difficulties that have to be solved to make its clinical application possible.
(Wu, 1998; Harvison, 2007).
Despite various obstacles, the position of streptokinase in the future is bright as the current tendencies of the market and the recent biotechnological methodology in the fields of reclaiming streptokinase reign over the difficulties of existing methods. These developments highlight two positive outcomes: an increase in the therapeutic effectiveness and safety of streptokinase, although the latter is a crucial factor in treatment and also helps in reducing the cost burden in resource-constrained settings. The present article will look into these issues critically giving promising developments about streptokinase and its most prevalent therapeutic directions, mainly in acute myocardial infarction and tissue plasminogen activator substitutions evolution (Yousaf, 2024).
Regulatory Considerations
Application in Medical Emergencies
The administration of the drug streptokinase intravenously gives the best outcome when used immediately. If attempted PCI cannot be started within 90-120 minutes, streptokinase drug comes to the rescue. Nevertheless, because of the development of immunity, its usage is generally limited to heart attack patients when they suffer from their first one (Gonzalez, 2020).
Variability and Market Availability
Promoted under the brand name such as Streptase, Mutose, Heberkinasa, STPase, and Myokinase streptokinase is licensed in various countries. It is produced by groups A, C, and G Streptococcus and consists of three domains: SK?, SK?, SK?, too. Upon looking into the ?-domain, it was found that it is a factor that affects the functionality of sK isoforms which is why a fibrin-specific variant that may work better and be safer might be developed (Rafipour, 2019).
The Antigenic Challenges in Streptokinase Usage
SK, a common thrombolytic drug used in heart diseases caused by blood thinning, has some anti-hapten immunogenic properties that react with patients' antibodies. This part discusses the immunogenicity challenges that the use of streptokinase is related to. It will further explain the identification of the antigenic regions and the strategies to overcome the immunogenicity (Kunamneni, 2006).
Identification of Antigenic Regions
Antigenic regions comprising streptokinase molecules are a key area to help us understand the possible modifications that can be applied to reduce immunogenicity. undefined
a) ?-DOMAIN (1–146 AMINO ACIDS):
Antigenic positions identified at 3-7, 4-8, 1-13, 1-20, 96-99, 138, and also regions 120-140 and 130-149.
b) ?-DOMAIN (147–290 AMINO ACIDS):
During the recognition and interaction at the 170-189, 1-253, and 238-246 sites, the plasminogen antigenic regions of this area provide high affinity for the Plg.
c) ?-DOMAIN (291–414 AMINO ACIDS):
Since it is an active site in very close proximity to the Plg domain, it contains immunogenic segments at positions 120–352, 390–399, 353–414, and 373–414 (Akbar, 2020).
Strategies to Reduce Immunogenicity
Efforts to decrease the immunogenicity of streptokinase have focused on modifying the structure of the molecule, particularly through truncation of certain regions:
? The 42 amino acid residues at the terminal section of the SK molecule when reduced by involvement in the immune system.
? One major common deletion, i.e., 1-59 amino acid, has been found to also decrease antigenicity. Besides, the plasminogen activator activity is also depressed.
? Among different truncations from the wild-type SK, for example, SK60-386 and SK143-386, it has been shown that all of them target the fibrinogen better, though decreasing the immunogenicity (Aghaeepoor, 2019).
Cost-Effectiveness and Scalability of Biotechnological Methods
Optimization and Production Efficiency
Biotech designations have tremendously increased and led to the production of Streptokinase employing the E.coli method. The use of RSM (Response Surface Methodology) for the optimization of the bio-engineered approach has enormously increased output by almost 4-fold. 14 times. Thus, yield can be specified as not just an indication of a potential improvement but as a factor that leads to low-cost generation processes (Aghaeepoor, 2019).
Economic Viability of Biotechnological Production
The lowest cost biotechnology after all traditional methods of producing streptokinase is when the biotechnology is compared. The contemporary modern biotechnology technique serves to speed up this process and to minimize to the minimum the cost of rehabilitative therapy, increasing its affordability. Also, along with a market price-benefit ratio deliberation that covers effective treatment efficiency and possible risks the economic health of these systems is supported (Ark, 1995).
Scalability of Production Methods
Scalability is an important parameter in biotechnology when it comes to the streptokinase production process. Scalability can be improved by fine-tuning the fermentation processes and refining downstream processing, which will in turn eventually lead to production efficiency. Efficient bioprocess designs are equally important as production plants on a large scale should guarantee good value and high quality of the streptokinase drug. The output from biotechnology production should be scalable to get the production needed for a large market without reducing its effectiveness (Bhardwaj, 2015).
Considerations for Large-Scale Production
The multifaceted nature of streptokinase means that this factor, at the industrial level, needs to be examined. This category involves capital investment needed for production facilities, operating costs involved, as well as demand expectation for the product in the target market. These kinds of analyses are necessary for identifying what the limitations of technologies are for mass production and if the cost will be absolutely viable when scaling up production.
Market and Manufacturing Costs
The market price of streptokinase at the end is made up of a number of factors such as materials and how the product comes to be. Investing in the efficient utilization of these costs is critical to ensure that streptokinase remains affordable. In low-resource settings, where the issues of high cost can hamper access to vital medicines, this becomes particularly important (Banerjee, 2004).
Biotechnological methods could yield game-changing results around the cost-service-effectiveness and scalability when it comes to skin ossification strategies, thus making these techniques more affordable and easily available, particularly in regions that are currently having to cope with very high costs of advanced medical treatment.
The development of Recombinant streptokinase production technology has changed traditional ways of enzymatic therapy for fibrinolysis, aimed at improving the therapeutic efficiency and reducing the underlying side effects.
Emerging Trends and Technologies in Recombinant Streptokinase Production Enhanced Production Techniques
Optimization of Recombinant Streptokinase Production in E. coli: Success was achieved by response surface methodology (RSM) optimization during IPTG production, the induction time after it, and the cell density at the onset of induction for the production of recombinant Synthetic Streptokinase. This optimized environment was the source of the 400% improvement. As a result of having a 14-fold greater SK production rate, we have a level of activity 615 less than the starting initial expression conditions of 1853 IU/mL. (Aghaeepoor, 2019b).
Cell-Free Production Systems: The last few years have seen great improvements in this area where cell-free systems produced some healthy streptokinase. These methods were as efficient and steady, with streptokinase yield of about 500 ?g/ml in a period of 2. 5 hours of speech, and allowed people to fully understand and express themselves. For example, the intein purification technique used in these systems gave us a recovery of 86% of the product, this implies that the finalized form of the recombinant streptokinase activities rose by about 86% (Tran, 2017).
Recombinant Streptokinase in Yeast Systems
Gene Cloning and Expression in Pichia pastoris: In the course of the experiment, the streptokinase gene was successfully cloned and made transcription into a eukaryotic expression vector. Further, the vector was then transformed into the yeast strain Pichia pastoris SMD1168. The final recombinant protein of streptokinase (rSK) was purified and showed very similar thrombolysis activity with the commercial one performing nearly 82% lysis of the blood clot at the concentration of 2000 U/ml.(Assiri, 2015).
Market Trends and Future Prospects
The recombinant streptokinase market segment is predicted to witness a rapid increase in the share due to the development of biotechnology and genetic engineering, the increasing prevalence of thrombotic disorders, and among others, the worldwide efforts at improving patient outcomes. The critical catalysts include new product innovations, allowing to target emerging markets, developing strategic partnerships, and conducting significant research and development.
These developments and innovations in the manufacturing and implementation of recombinant fibrinolytic progress not only improve its pharmacologic effect but also promise to facilitate the availability and economic effectiveness of thrombolytic therapy in regions where the burden of thromboembolic diseases is extensive.
Future Market Outlook
The global market of Streptokinase is expected to see high-level growth from 2023 to 2030 primarily due to a wider spread of cardiovascular diseases and an enlarging number of elderly people. The market dynamism is shaped by factors such as cutting-throat competition, fast technological advancements, and the need to fit customer demands. Of all the participants in the industry, Samarth Pharma, Biocon, and KeePharma are the main ones (Dataintelo, 2023).
The section also demonstrates the learnings from the Streptokinase therapy evolving landscape and emphasizes the continued need for innovation and strategic adaptations within the market to enhance the efficiency and availability of thrombolytics treatments.
Conclusion and Recommendations for Streptokinase Development
While we have delved into the various facets of streptokinase therapy such as its production, its antigenicity the recombinant technology as well as its market growth, the key message remains plain. The history of streptokinase therapy, from the findings of fundamental research to the challenges patients had to deal with, is telling of how complicated biotechnological improvement is and how the application in the clinic is taking shape. Even analyzing how far forward we've come about in improving the efficiency, safety, and accessibility of this all-important thrombolytic agent will serve as a solid foundation for our future research and development efforts.
In the future, Streptokinase treatment's road to navigate the impact of biotechnology and medicine advancements, and eventually, improve the outcomes for patients with thrombotic illnesses. Due to the development of that area, recognition of current problem solving through novel research, adding market analysis, and patient orientations will be the way for improved, available, and cost-effective fibrinolytic therapy. Reflecting on that not only demonstrates the need for progress in medical science but also pinpoints that exploration and adaptation are always relevant in the world of unpredictable medical science.
Our expedition has covered the intricate territory of streptokinase therapy, the fundamental role of which is to treat thromboembolic conditions and overcome the biotechnological problems associated with it. The talk highlighted the important breakthroughs in recombinant streptokinase production, DNA modifications for minimizing immunogenicity, and cost-effectively producible scalable methods. Through these efforts, streptokinase's clinical efficacy may further be enhanced and its accessibility increased amidst a rapidly aging world and growing cardiovascular disease' prevalence. Such a development cannot be underestimated - indeed it sets the stage for more precise and safer streptokinase therapy, more widely accessible to those who are in need.
The market projections for streptokinase are a panorama of growth and creation, with recombinant technology being seen as the main player in tackling the problem of immunogenicity and production inefficiencies. Emphasis on multimodal therapy, initiation of the exploration of alternative delivery methods, and strategic consideration of the possible existence of market and clinical barriers is a collaborative effort that will lead to optimal usage of streptokinase. This therapy is at a crossroads in terms of its implementation and innovation processes. However, the future of streptokinase seems brighter than ever in terms of its establishing a leading position in the medical field and enhancing its life-saving prospects on an international scale.
References
-
Adayon, A. R., & Jamshidi, A. (2015). Esmaeili, Delivery of tissue plasminogen activator and streptokinase magnetic nanoparticles to target vascular diseases. International journal of pharmaceutics. 495(1): p. 428-438.
- Aghaeepoor, M., Akbarzadeh, A., Kobarfard, F., Shabani, A. A, Dehnavi, E., Jamshidi, A. S., & Akbari, E. M. R. (2019). Optimization and High Level Production of Recombinant Synthetic Streptokinase in E. coli Using Response Surface Methodology. Iran J Pharm Res. Spring;18(2), 961-973. https://doi.org/10.22037/ijpr.2019.1100636.
-
Aghaeepoor, M., et al., (2017). Optimization of culture media for extracellular expression of streptokinase in Escherichia coli using response surface methodology in combination with Plackett-Burman Design. Tropical Journal of Pharmaceutical Research, 16(11): p. 2567-2576.
- Aguilera, A., et al., (2013). Formulation development of a recombinant Streptokinase suppository for hemorrhoids treatment. Biotecnología Aplicada, 30(3): p. 182-186.
- Assiri, A. S, El-Gamal, B. A., Hafez, E. E., & Haidara, M.A. (2014). Production of recombinant streptokinase from Streptococcus pyogenes isolate and its potential for thrombolytic therapy. Saudi Med J. 35(12): 1482-8.
- Babashamsi, M. M. H., & Razavian, M. R. (2009). Nejadmoghaddam, Production and Purification of Streptokinase by Protected Affinity Chromatography. Avicenna Journal of Medical Biotechnology, 1(1), 47-51.
- Battershill P. E., Benfield, P., & Goa, K. L. (1994). Streptokinase. A review of its pharmacology and therapeutic efficacy in acute myocardial infarction in older patients. Drugs Aging, 4(1):63-86. https://doi.org/10.2165/00002512-199404010-00007
-
Betancourt, B. Y., et al. (2005). Pharmacovigilance program to monitor adverse reactions of recombinant streptokinase in acute myocardial infarction. BMC Clinical Pharmacology, 5: p. 1-7.
- Deepika, G., Girish, S., & Debendra K. S. (2009). Enhanced production of recombinant streptokinase in Escherichia coli using fed-batch culture, Bioresource Technology, 100(19), 4468-4474, https://doi.org/10.1016/j.biortech.2009.04.008
-
Ehab, E. D., Hend, O., Safia S., Sami, M. N., Hadeer A. E. K., & Mohamed, A. S. (2022). Optimization of high expression and purification of recombinant streptokinase and in vitro evaluation of its thrombolytic activity, Arabian Journal of Chemistry, 15(5).
https://doi.org/10.1016/j.arabjc.2022.103799 -
Gonzalez, P. E., Omar, W., Patel, K., De Lemos, J. A., Bavry, A. A., Koshy, T., Mullasari, A., Alexander, T., Banerjee, S., & Kumbhani, D. J. (2020). Fibrinolytic strategy for ST-Segment–Elevation myocardial infarction. Circulation. Cardiovascular Interventions, 13(9).
). https://doi.org/10.1161/circinterventions.120.009622 -
Hagenson, M. et al. (1989). Expression of streptokinase in Pichia pastoris yeast. Enzyme and microbial technology, 11(10): p. 650-656.
- Harvison, P. J. (2007). Streptokinase. In Elsevier eBooks (1–6). https://doi.org/10.1016/b978-008055232-3.62677-7
-
Jin, S. E., Kim, I. S., & Kim, C. K. (2015). Comparative effects of PEG-containing liposomal formulations on in vivo pharmacokinetics of streptokinase. Archives of pharmacal research, 38: p. 1822-1829.
-
Karimi, Z., et al., (2011). Fermentation, fractionation and purification of streptokinase by chemical reduction method. Iranian Journal of Microbiology, 3(1): p. 42.
- Lee, B. R., et al., (1989). Site-specific alteration of Gly-24 in streptokinase: its effect on plasminogen activation. Biochemical and biophysical research communications, 165(3): p. 1085-1090.
- Locke, M., et al., (2020). An international collaborative study to establish the WHO 4th International Standard for Streptokinase: Communication from the SSC of the ISTH. Journal of Thrombosis and Haemostasis, 18(6): 1501-1505
- Longstaff, C., Thelwell, C., & Whitton. (2005). The poor quality of streptokinase products in use in developing countries, Journal of Thrombosis and Haemostasis, 3(5), 1092-1093, https://doi.org/10.1111/j.1538-7836.2005.01271.x.
-
Malke, H., & Ferretti, J. J. (1984). Streptokinase: cloning, expression, and excretion by Escherichia coli. Proceedings of the National Academy of Sciences, 81(11): p. 3557-3561.
- Modaresi, S. M. S., et al., (2014). Preparation and characterization of self-assembled chitosan nanoparticles for the sustained delivery of streptokinase: an in vivo study. Pharmaceutical development and technology, 19(5): p. 593-597
- Monk, J. P., & Heel, R. C. (1987). Anisoylated Plasminogen Streptokinase Activator Complex (APSAC). Drugs 34, 25–49. https://doi.org/10.2165/00003495-198734010-00002
-
Perkins, W., et al., (1997). Streptokinase entrapment in interdigitation-fusion liposomes improves thrombolysis in an experimental rabbit model. Thrombosis and haemostasis, 77(06): 1174-1178.
- Pimienta, E., Ayala, J.C., Rodríguez, C. et al. (2007). Recombinant production of Streptococcus equisimilis streptokinase by Streptomyces lividans. Microb Cell Fact 6, 20.
-
Pimienta, E., et al. (2007). Recombinant production of Streptococcus equisimilis streptokinase by Streptomyces lividans. Microbial Cell Factories, 6: p. 1-8.
- Pitek, A. S., et al., (2017). Elongated plant virus-based nanoparticles for enhanced delivery of thrombolytic therapies. Molecular pharmaceutics, 14(11): p. 3815-3823.
-
Pitek, A. S., et al., (2018). Delivery of thrombolytic therapy using rod-shaped plant viral nanoparticles decreases the risk of hemorrhage. Nanoscale, 10(35): p. 16547-16555.
- Sobel, B. E. (1987). Safety and Efficacy of Tissue-Type Plasminogen Activator Produced by Recombinant DNA Technology. SAFETY, 40: p. 4B.
- Sohaimy, S., et al., (2011). Expression of recombinant Streptokinase from local Egyptian Streptococcus sp. SalMarEg. African Journal of Biotechnology, 10(45): p. 9001-9011.
- Vaidya, B., et al., (2016). Development and characterization of highly selective target-sensitive liposomes for the delivery of streptokinase: in vitro/in vivo studies. Drug delivery, 23(3): p. 791-797.
- Vellanki, R. N. et al. (2013). Constitutive optimized production of streptokinase in Saccharomyces cerevisiae utilizing glyceraldehyde 3-phosphate dehydrogenase promoter of Pichia pastoris. BioMed Research International,
- Wu, X., Ye, R., Duan, Y., & Wong, S. L. (1998). Engineering of plasmin-resistant forms of streptokinase and their production in Bacillus subtilis: streptokinase with longer functional half-life. Appl Environ Microbiol. 64(3):824-9.
- Yaghoobi, N., et al., (2017). Preparation, optimization and activity evaluation of PLGA/streptokinase nanoparticles using electrospray. Advanced Pharmaceutical Bulletin, 7(1): p. 131.
- Yousaf, S., Arshad, M., Harraz, F. A., Masood, R., Zia, M. A., Jalalah, M., & Faisal, M. (2024). Evaluation of clinical efficacy of streptokinase by comparison with the thrombolytic agent on animal model. Braz J Biol. 26, 84:e271083. https://doi.org/10.1590/1519-6984.271083.
- Zhang, X., et al. (1999). Recombinant streptokinase production by fed-batch cultivation of Escherichia coli. Enzyme and Microbial Technology, 24(10): p. 647-650
- Rafipour, M., Keramati, M., Aslani, M. M., Arashkia, A., & Roohvand, F. (2019). The β-domain of streptokinase affects several functionalities, including specific/proteolytic activity kinetics. FEBS Open Bio. 9(7):1259-1269. https://doi.org/10.1002/2211-5463.12657.
- Kunamneni, A., Abdelghani, T. T. A., & Ellaiah, P. (2006). Streptokinase—the drug of choice for thrombolytic therapy. Journal of Thrombosis and Thrombolysis, 23(1), 9–23. https://doi.org/10.1007/s11239-006-9011-x
-
Akbar, G., Zia, M. A., Ahmad, A., Arooj, N., & Nusrat, S. (2020). Review on Streptokinase with its Antigenic Determinants and Perspectives to Develop its Recombinant Enzyme with Minimum Immunogenicity. Journal of Innovative Sciences, 6(1). https://doi.org/10.17582/journal.jis/2020/6.1.17.23
https://doi.org/10.17582/journal.jis/2020/6.1.17.23 - Aghaeepoor, M., Akbarzadeh, A., Kobarfard, F., Shabani, A. A., Dehnavi, E., Aval, S. J., & Eidgahi, M. R. A. (2019). Optimization and High Level Production of Recombinant Synthetic Streptokinase in E. coli Using Response Surface Methodology. PubMed, 18(2), 961–973. https://doi.org/10.22037/ijpr.2019.1100636
- Ark, A., H., Latky, Obert, M., C., Aliff, C., D., Avid, Aylor, Erry, L., L., Ee, Aul, W., A., Rmstrong, Abriel, Arbash, Harvey, Hite, Aarten, L., S., Imoons, Charlotte, L., N., Elson, Ancy, Lapp, Hanning, J., D., Night, Rank, E., H., Arrell, Ohn, Imes, Ric, J., T., & Opol. (1995). Cost effectiveness of thrombolytic therapy with tissue plasminogen activator as compared with streptokinase for acute myocardial infarction. The New England journal of medicine, 332 21, 1418-24.
- Bhardwaj S., & Angayarkanni J. (2015). Streptokinase production from Streptococcus dysgalactiae subsp. equisimilis SK-6 in the presence of surfactants, growth factors and trace elements. 3 Biotech. 5(2):187-193. https://doi.org/10.1007/s13205-014-0209-x. . https://doi.org/10.1007/s13205-014-0209-x
- Aghaeepoor, M., Akbarzadeh, A., Kobarfard, F., Shabani, A. A., Dehnavi, E., Aval, S. J., & Eidgahi, M. R. A. (2019b). Optimization and High Level Production of Recombinant Synthetic Streptokinase in E. coli Using Response Surface Methodology. PubMed, 18(2), 961–973. https://doi.org/10.22037/ijpr.2019.1100636 https://doi.org/10.22037/ijpr.2019.1100636
- Tran, K., Gurramkonda, C., Cooper, M. A., Pilli, M., Taris, J. E., Selock, N., Han, T. C., Tolosa , M., Zuber, A., Peñalber-Johnstone, C., Dinkins, C., Pezeshk, N., Kostov, Y., Frey, D. D., Tolosa, L., Wood, D. W., & Rao, G. (2018). Cell-free production of a therapeutic protein: Expression, purification, and characterization of recombinant streptokinase using a CHO lysate. Biotechnol Bioeng. 115(1):92-102. https://doi.org/10.1002/bit.26439. . https://doi.org/10.1002/bit.26439
- Assiri, A. S., El-Gamal, B. A., Hafez, E. E., & Haidara, M. A. (2014). Production of recombinant streptokinase from Streptococcus pyogenes isolate and its potential for thrombolytic therapy. Saudi Med J. 35(12), 1482-8.
-
Dataintelo, S. R., & Dataintelo. (2023, September 2). Streptokinase Market Report | Global Forecast from 2023 to 2032. Dataintelo. https://dataintelo.com/report/global-streptokinase-market/. https://dataintelo.com/report/global-streptokinase-market/
-
https://dataintelo.com/report/global-streptokinase-market/Renzo, P. K., Siiteri, B. L., & Hutchings, P. H. (1967). Bell, Preparation and Certain Properties of Highly Purified Streptokinase, Journal of Biological Chemistry, 242(3), 533-542, https://doi.org/10.1016/S0021-9258(18)96306-4.https://doi.org/10.1016/S0021-9258(18)96306-4.
- Ghosh, S., Saha, S., & Sahoo, S. (2021). Production of Thrombolytic and Fibrinolytic Proteases: Current Advances and Future Prospective. In: Thatoi, H., Mohapatra, S., Das, S.K. (eds) Bioprospecting of Enzymes in Industry, Healthcare and Sustainable Environment. Springer, Singapore. https://doi.org/10.1007/978-981-33-4195-1_17. https://doi.org/10.1007/978-981-33-4195-1_17.
Cite this article
-
APA : Asim, M., Mushtaq, F., & Qadeer, M. (2023). Biotechnological Advancements in Streptokinase Production. Global Drug Design & Development Review, VIII(IV), 46-60. https://doi.org/10.31703/gdddr.2023(VIII-IV).05
-
CHICAGO : Asim, Muhammad, Faiza Mushtaq, and Minahil Qadeer. 2023. "Biotechnological Advancements in Streptokinase Production." Global Drug Design & Development Review, VIII (IV): 46-60 doi: 10.31703/gdddr.2023(VIII-IV).05
-
HARVARD : ASIM, M., MUSHTAQ, F. & QADEER, M. 2023. Biotechnological Advancements in Streptokinase Production. Global Drug Design & Development Review, VIII, 46-60.
-
MHRA : Asim, Muhammad, Faiza Mushtaq, and Minahil Qadeer. 2023. "Biotechnological Advancements in Streptokinase Production." Global Drug Design & Development Review, VIII: 46-60
-
MLA : Asim, Muhammad, Faiza Mushtaq, and Minahil Qadeer. "Biotechnological Advancements in Streptokinase Production." Global Drug Design & Development Review, VIII.IV (2023): 46-60 Print.
-
OXFORD : Asim, Muhammad, Mushtaq, Faiza, and Qadeer, Minahil (2023), "Biotechnological Advancements in Streptokinase Production", Global Drug Design & Development Review, VIII (IV), 46-60
-
TURABIAN : Asim, Muhammad, Faiza Mushtaq, and Minahil Qadeer. "Biotechnological Advancements in Streptokinase Production." Global Drug Design & Development Review VIII, no. IV (2023): 46-60. https://doi.org/10.31703/gdddr.2023(VIII-IV).05